The next step might be to create an autonomous assembly line, just like in biology where independent factories, or "ribosomes", produce different proteins according to the chemical messages. But also the decay chain must be created? Nanoparticles free in nature may be dangerous.
"Eventually we want to make something as complex as a cell that can have all these independent little factories running in it, each one cranking out a different product based on their program – and this is what Seeman's paper is starting to show us how to do," says Rothermund.
Self-assembly can combine and build up assemblies efficiently, but may be subject to thermodynamic and kinetic limitations: reactants, intermediates and products may collide with each other throughout the assembly time course to produce non-target species instead of target species. An alternative approach to nanoscale assembly uses information-containing molecules such as DNA to control interactions and thereby minimize unwanted cross-talk between different components. Maybe Nature itself had come to the same solution? We can build an car on an assembly line? (This is an often used claim in religious debates - it isn't possible. So they are wrong.)
Two Nature articles (1,2) from may this year.
New insights and breakthroughs:
1. Nanorobots: DNA robots mimic the protein motors in our bodies – be it walking without help along predefined routes or taking cargo from A to B. This combine advances in our knowledge of DNA structure and dynamics, suggest that nanorobots could soon be performing autonomous, useful tasks.
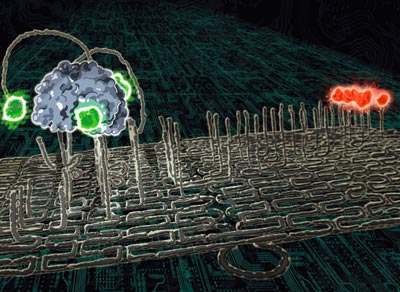
1.a. DNA walkers, have a body and feet made from DNA, with extra "anchor" strands of DNA that join the feet to a surface. When different "fuel" strands are put in front of a walker, they preferentially join to the anchor strands, thereby freeing the walker to move forward. Milan Stojanovic. The spider moving forwards in a straight line leaving a trail of cleaved DNA (or cut grass) behind. "An observer, looking at it, could legitimately say that the molecule 'behaves' in a certain way, although in reality the spider just implements simple leg residency rules." The process is fully autonomous.
1.b. Molecular spider, has a protein body and DNA legs that chemically cleave tall strands of DNA on a surface. Simply put, a molecular spider nibbles its way through a lawn of DNA, only going where there is more grass to mow. Ned Seeman, a passing walker can take on eight (that is, 2^3) possible loads and deposit them at the end of the track. The walker, the three independently programmed addition stations and the cargo are all sitting on a DNA origami platform. It is the first assembly line built on the nanometre scale.
Solution: "DNA origami". Based on folded DNA, can be realized by the judicious combination of three known DNA-based modules; makes something akin to a pegboard to which all sorts of molecules can be attached. Folding DNA to create nanoscale shapes and patterns. Paul W. K. Rothemund.
The informational problem.
Robots have to store lots of information in order to coordinate their actions, but how can this be done for nanometre-scale robots? One answer is to program data into the robots' environment instead. Traditional robots rely for their function on computing, to store internal representations of their goals and environment and to coordinate sensing and any actuation of components required in response. Moving robotics to the single-molecule level is possible in principle, but requires facing the limited ability of individual molecules to store complex information and programs. One strategy to overcome this problem is to use systems that can obtain complex behaviour from the interaction of simple robots with their environment.
“Carbon nanotubes will be one of the major future building blocks of molecular electronics due to their small dimensions and excellent electronic properties,” said Braun. “However, you cannot self-assemble a circuit directly with nanotubes because they lack recognition. Science 302 1380
When origami templates and DNA-functionalized nanotubes are mixed, strand displacement-mediated deprotection and binding aligns the nanotubes into cross-junctions. Of several cross-junctions synthesized by this method, one demonstrated stable field-effect transistor-like behaviour. In such organizations of electronic components, DNA origami serves as a programmable nanobreadboard; thus, DNA origami may allow the rapid prototyping of complex nanotube-based structures.
DNA and superconduction.
DNA molecules act as ohmic conductors above 1 K and below this temperature they can superconduct (A Y Kasumov et al 2001 Science 291 280). Carbon nanotubes rolled up sheets of graphite atoms lose their resistance when connected to superconductors. Now Kasumov has shown that this is also true for DNA by connecting double-stranded DNA molecules to rhenium and carbon superconducting electrodes 0.5 µm apart. By cooling the electrodes to below their superconducting transition temperatures, the researchers observed so-called 'proximity induced' superconductivity in the DNA.
Optical experiments have shown that a transfer of charge may be possible in such molecules. But the message from transport measurements has been mixed: some have indicated that DNA could be a conductor while others suggested that DNA is an insulator. Kasumov and colleagues have found that above 1 K, the resistance per molecule is less than 100 kilo-ohm, a figure that varies weakly with temperature and is an order of magnitude lower than previous measurements. Even at very low temperatures, the researchers found that DNA molecules can conduct ohmically over distances of a few hundred nanometres.
Pi-stacks have been shown to conduct charge. Stacking in supramolecular chemistry refers to a stacked arrangement of often aromatic molecules, which is adopted due to interatomic interactions. The most common example of a stacked system is found for consecutive base pairs in DNA. Stacking also frequently occurs in proteins where two relatively non-polar rings overlap. Stacking is often referred to as π-π interaction, though effects due to the presence of a π-orbital are only one source of such interactions, and in many common cases appear not to be the dominant contributors. an aromatic interaction (or π-π interaction) is a noncovalent interaction between organic compounds containing aromatic moieties. π-π interactions are caused by intermolecular overlapping of p-orbitals in π-conjugated systems, so they become stronger as the number of π-electrons increases. Other noncovalent interactions include hydrogen bonds, van der Waals forces, charge-transfer interactions, and dipole-dipole interactions. In DNA, pi stacking occurs between adjacent nucleotides and adds to the stability of the molecular structure. The nitrogenous bases of the nucleotides are made from either purine or pyrimidine rings, consisting of aromatic rings. Within the DNA molecule, the aromatic rings are positioned nearly perpendicular to the length of the DNA strands. Thus, the faces of the aromatic rings are arranged parallel to each other, allowing the bases to participate in aromatic interactions. The bases in the middle of the double helix stack in a way reminiscent of graphite – which is an excellent conductor. Through aromatic interactions, the pi bonds, extending from atoms participating in double bonds, overlap with pi bonds of adjacent bases. This is a type of non-covalent chemical bond. Though a non-covalent bond is weaker than a covalent bond, the sum of all pi stacking interactions within the double-stranded DNA molecule creates a large net stabilizing energy.
Also the DNA backbone – the long strands that support the bases and give DNA its structure – rather than the bases, might support conduction because of its periodic structure. Conduction in terms base stacking have yielded conflicting results. Alternative or complementary conduction mechanisms – such as Brillouin’s backbone conduction – have been largely ignored ( (Phys. Rev Lett. 99 228102 ). Electrons in the backbone delocalize in less than one femtosecond (10-15) in wet DNA. These results imply that electron movement occurs a thousand times faster in the DNA backbone than in the bases stacked in the core. Focusing on the interplay between electron transport through the backbone and the stacked bases could be crucial to understanding DNA conduction.
A better understanding of the role that conduction plays in how living cells detect and repair damaged DNA and could ultimately lead to strands of DNA being used in “molecular electronics” technologies of the future. Conduction is thought to be an important mechanism by which enzymes recognize damaged DNA. Conduction through DNA could protect the genomes of some organisms by transmitting the damage caused by oxidizing chemicals to certain locations on chromosomes where the damage causes the least harm. This is the mitogenic signal for negative energy pray?
It is possible that the contacts act as strong dopants of electrons or holes. Conductivity measurements could in turn help biologists to look for particular sequences of base pairs within DNA molecules.
Without carbon - no Life.
Carbon is a composite boson and essential to Life. Graphene, for example, possesses very uncommon electronic structure and a high carrier mobility, with charge carriers of zero mass moving at constant velocity, just like photons. Future prospects, difficulties and challenges with graphene include growth, morphology, atomic and electronic structure, transport properties, superconductivity, doping, nanochemistry using hydrogen, chemical and bio-sensors, and bio-imaging etc.
Also single sheets of graphane, that is graphene functionalized upon hydrogen, are investigated. At full H-coverage nearly free electron states (NFESs) appear near the conduction band minimum in all the systems under study. At the same time, the electron affinity is strongly reduced becoming negative for the hydrogenated diamond surfaces, and almost zero in graphane. The effects of quasi-particle corrections on the electron affinity and on the NFESs are discussed.
Carbon has the biggest numbers of isomeric states, eight in total.
Magnetism, time and temperature.
The magnetization direction can become unstable due to thermal fluctuations. Then, the magnetization fluctuates randomly between two stable directions. This phenomenon is called superparamagnetism. It constitutes one of the greatest limit to the increase of magnetic memories storage density. Memory needs time.

Energy level diagram describing the stabilization of the ferromagnetic spin arrangement as a result of the interaction between two hybrid orbitals located on two impurities, here labeled TM1 and TM2, in a tetrahedral semiconductor.
Two proactive cultures of predictive theories have emerged:
A. The initial basic philosophy was to use model Hamiltonians, in which certain specific magnetic interactions between ions are postulated (and others are excluded). For this technique, the “user” needs to guess at the outset the type of magnetic interactions that will dominate. This style of model-Hamiltonian theory has had a glorious past in numerous areas of condensed matter physics. It was assumed it was similar to the interaction of nuclear spins mediated by the conduction electrons in metals, and the model also assumes that the host crystal is largely unperturbed by the presence of the 3d impurity ion, and also predict ferromagnetism in various 3d impurity-doped compound semiconductors, among all the prediction that ferromagnetism persists to a high Curie temperature with TC’s well above room temperature in various wide-gap oxides and nitrides.
In a ferromagnet there is an imbalance in the occupancy of spin-up and spin-down states; such a collective spin alignment leads to a macroscopic spontaneous magnetization that persists up to a Curie temperature TC. Many common ferromagnets (such as iron or nickel) are metals, exhibiting a set amount of free electrons that make them conduct electrically. In semiconductors, on the other hand, the concentration and type of electronic carriers is controllable externally, for instance, by doping with select atom types or directly by injecting carriers. Most semiconductors are not magnetic. A material that exhibits both ferromagnetic and semiconductor properties offers the exciting prospect of combining nonvolatile magnetic storage and conventional semiconductor electronics in a single device. Magnetic semiconductors offer a number of interesting possibilities in the pursuit of “spintronics,”a branch of science and technology that exploits the spin dimension of the electron in addition to its charge, for novel electronic devices. These materials combine the properties of a semiconductor and a magnetic material, providing, for instance, a way to create 100% spin-polarized currents, and by the same token, the promise of electrical control of magnetic effects.
Nonmagnetic semiconductor host materials can be doped by a small amount of magnetic transition-metal ions or by defects that promote magnetism (“dilute magnets”). When doped with manganese, Mn2+ substitute for Ga3+ ions, thus releasing “holes” (a positive charge resulting from the absence of an electron) that are said to collectively align the Mn2+ spins ferromagnetically when the sample is cooled below TC.
The description of the superparamagnetic phenomenon has been for a long time simplified by averaging the atomic moments in the particle so that any internal degree of freedom and excitations are then neglected in old model. We have developed a new model, which restores all the internal degree of freedom, using an atomic description and including specifically the temperature. This model has shown that spin-wave eigenmodes can be excited by the temperature and induce an increase of the superparamagnetic fluctuations in the magnetic nanoparticles.
B. First-principles calculations have rapidly penetrated this field. The philosophy here is to describe each host and impurity system by considering its fundamental electronic structure, thereby obtaining the magnetic interaction type as the answer, not the question. Instead of prejudging the preferred type of magnetic interactions between ions, one articulates fundamental electron-ion and electron-electron interactions and lets the magnetic interactions emerge as solutions to the fundamental many-electron Hamiltonian. The availability of density-functional approximations (exchange and correlation functionals for the inter-electronic interactions), accurate pseudopotentials (simplifying the calculation greatly), and the ubiquitous computer packages encoding such developments into friendly interfaces freed the theorist from having to guess at the outset how a particular impurity would affect a particular host crystal.
Nuclear spin and electron spin make the magnetic pattern necessary for chemical movement and energy movement. Impurities direct and strengthen, as GABA inhibits and glutamat strengthen. Impurities are as necessary as noise are in biology.
Fractality and bond entanglements.Fractal patterns are ubiquitous in nature from the shape of a galaxy to the structure of an atom/particle. They may also lie behind the mysterious phenomenon of high-temperature superconductivity; high-temperature superconductors do not appear to create the pairs of electrons needed for zero-resistance conductivity via vibrations of the crystal lattice. suggested that high-temperature superconductivity may be linked to the distribution of oxygen ions in the layers between the copper oxide. They say it might be the formation of some of these ions into rows, or "stripes", that is responsible.
What they found was that this intensity followed a power-law distribution, in other words that the superconductor was made up of a small number of very high-ordered regions and larger numbers of disordered regions. This, they say, is the hallmark of a scale-free distribution, which is typical of a fractal pattern – with the oxygen stripes forming a similar structure on all scales up to 400 µm.
Partial density of states in the empty conduction band of the phosphate backbone sites in DNA?
Role of the γ-phosphate of ATP in triggering protein folding?
Well-matched duplex DNA in the gap between the electrodes exhibits a resistance on the order of 1 M


Structural and functional analysis of condensed DNA Nucleosome core particles self organise into multiple crystalline or liquid crystalline phases. Nucleosome stack on top of each other to form columns. These columns further organise into multiple crystalline or liquid crystalline phases. A lamello-columnar and an inverse hexagonal phase form under low salt conditions. A 2D hexagonal and a 3 D orthorhombic phase are found at higher salt concentrations.



Walking molecules and oxygen.
Molecular machines could be capable of quantum mechanical tunnelling - normally seen in very tiny particles like electrons and atoms. The tunnelling behaviour has never before been seen in devices so big and is a fundamental departure from mechanics in the macroscopic world, says Ludwig Bartels. It also means that such machines could move much faster than expected.
Bartels and colleagues found that anthraquinone could walk across copper surfaces in a straight line. This was an important result in itself because normal molecules tend to move around randomly in all directions. Moreover, anthraquinone can attach carbon dioxide molecules to its two oxygen atoms, or "legs", and drag this cargo along too while it walks. However, the molecule moved so fast.
Pentacenetetrone has not two oxygen "legs" as in anthraquinone but four. To their surprise, Bartels & co found that this "quadrupedal" molecule, which moves like a pacing horse (both legs on one side of the molecule move together followed next by the two legs on the other side), moved a million times slower than "bipedal" anthraquinone. One foot can just start tunnelling forward at any time and make a successful step.
Portions of the bipedal anthraquinone simply tunnel through barriers (like surface roughness or corrugations) in the environment rather than climbing over them. Although the quadrupedal molecule can coordinate its four "hooves" so that it paces forward, it cannot coordinate both legs so that they tunnel through a surface barrier at the same time. This means that the molecule needs to move its oxygen legs in the conventional way – over the barriers.
Quantum mechanics allows such behaviour for very light particles, like electrons and hydrogen atoms but could it also be relevant for big molecules like anthraquinone?
Molecule Diffusion through Tunneling versus Pacing.
The diffusion temperature of molecular ‘walkers’, molecules that are capable of moving unidirectionally across a substrate violating its symmetry, can be tuned over a wide range utilizing extension of their aromatic backbone, insertion of a second set of substrate linkers (converting bipedal into quadrupedal species), and substitution on the ring. Density functional theory simulation of the molecular dynamics identifies the motion of the quadrupedal species (through electric structure of matter) as pacing (as opposed to trotting or gliding). Knowledge about the diffusion mode allows us to draw conclusions on the relevance of tunneling to the surface diffusion of polyatomic organic molecules.
Why bother? The class of molecules investigated here can transport themselves and molecular cargo on an unpatterned surface in a predictable fashion. Directed transport of molecules is fundamental to life: for instance, kinesin motor proteins transport vesicles and organelles to their point of deployment inside a cell.
Fermi surfaces.
High temperature superconductors is highly anisotropic. The Fermi surface is very close to the Fermi surface of the doped CuO2 plane (or multi-planes, in case of multi-layer cuprates) and can be presented on the 2D reciprocal space (or momentum space) of the CuO2 lattice. The typical Fermi surface within the first CuO2 Brillouin zone is sketched below.In a wide range of charge carrier concentration (doping level), in which the hole-doped superconductor, the Fermi surface is hole-like (i.e. open).

Solids with a large density of states at the Fermi level become unstable at low temperatures and tend to form ground states where the condensation energy comes from opening a gap at the Fermi surface. Examples of such ground states are superconductors, ferromagnets, Jahn-Teller distortions and spin density waves.
The state occupancy of fermions like electrons is governed by Fermi-Dirac statistics so at finite temperatures the Fermi surface is accordingly broadened. In principle all fermion energy level populations are bound by a Fermi surface
In condensed matter physics, the Fermi surface is an abstract boundary useful for predicting the thermal, electrical, magnetic, and optical properties of metals, semimetals, and doped semiconductors. The existence of a Fermi surface is a direct consequence of the Pauli exclusion principle. the particles fill up all energy levels till εF, which is equivalent to saying that εF is the energy level below which there are exactly N states.
In momentum space, these particles fill up a sphere of radius pF, the surface of which is called the Fermi surface.
The linear response of a metal to an electric, magnetic or thermal gradient is determined by the shape of the Fermi surface, because currents are due to changes in the occupancy of states near the Fermi energy. Free-electron Fermi surfaces are spheres determined by the valence electron concentration where \hbar is the reduced Planck's constant. When a material's Fermi level falls in a bandgap, there is no Fermi surface. As with the band structure itself, the Fermi surface can be displayed in an extended-zone scheme where \vec{k} is allowed to have arbitrarily large values or a reduced-zone scheme. Fermi surfaces have been measured through observation of the oscillation of transport properties in magnetic fields. The oscillations are periodic versus 1 / H and occur because of the quantization of energy levels in the plane perpendicular to a magnetic field, a phenomenon first predicted by Lev Landau. The new states are called Landau levels and are separated by an energy \hbar \omega_c where ωc = eH / m * c is called the cyclotron frequency, e is the electronic charge, m * is the electron effective mass and c is the speed of light.
With positron annihilation the two photons carry the momentum of the electron away; as the momentum of a thermalized positron is negligible, in this way also information about the momentum distribution can be obtained. Because the positron can be polarized, also the momentum distribution for the two spin states in magnetized materials can be obtained.

Update jan. 2014:
Mitochondrial transfer from stem cells to damaged cells to help them heal.Epithelial mitochondrial dysfunction is critical in asthma pathogenesis. Here we show for the first time that Miro1, a mitochondrial Rho‐GTPase, regulates intercellular mitochondrial movement from mesenchymal stem cells (MSC) to epithelial cells (EC). We demonstrate that overexpression of Miro1 in MSC (MSCmiroHi) leads to enhanced mitochondrial transfer and rescue of epithelial injury.
Wish you would find the energy to continue.
SvaraRaderaUlla, a great post and a beautiful picture.
It inspired a coming together of some ideas today I think you can appriciate it and like the alternative view. See my post The Synthetic Engine.
The PeSla pesla.blogspot.com
You know, I have some figures on my mind. Hexagons are one. Also the important building blocks of Life, oxygen and hydrogen, metals, and their relations. If you put a carbon atom in each corner of the Fermi surface you have an important piece, modulated by methane. Why is it done in that way?
SvaraRaderaIn sugars you get instead an pentagon. It is about forms as essential structures.
Ulla
SvaraRaderaOf course the hexagons are part of the diamond lattice- unless they are flat thus zero probability and organic aromatic principles (also found on buckyball surfaces)
For my connection with Fermi surfaces I offer you only the photo of my blog on
http://4.bp.blogspot.com/_UF6vrnsBd3U/S4q-6V6W1LI/AAAAAAAAAaM/k8U6iIMS5_A/s1600-h/supersympx.jpg to show the geometry- but the depth of my understanding then is a little obsolete.
Rowlands points out in DNA there can be alternative ways to stack it with four ways to understand where the sugar goes
Nitrogen and in NH3 could certainly be a source of quantum flux in a mental or organic system or between synapses according to Wolfe.
The vibration state if a carbon atom (methane and so on) has 15 possible ones observed. Where is the 16th (presumed superimposed)? But I see this as a matter of geometry and Venn logic.
On should really see a carbon like structures as the five points of a four dimensional simplex (made of triangles).
Such ideas may be had to see but easy to intuit so pardon if we are not on the same partical length :-) your interest in all this impresses me very much.
This link may be of general interest to you too.
http://pesla.blogspot.com/2010/04/casual-encounter-with-peter-rowlands.html
I guess I should index stuff here as it was so much to try to find, but pictures help.
ThePeSla
Nitrogen is surely a quantum gas. Look at the effects of NO :)
SvaraRaderaThe different atoms and molecules form a network of negentropy, meaning (reduction) that is broken (oxidation).
As I said Rowland has bad foundations. otherwise his thoughts are good, I think.
I should index, I know. I started in swedish, then I noticed I must write in english and so it left. Sry.
http://emboj.embopress.org/content/early/2014/01/15/embj.201386030
SvaraRaderaMiro1 regulates intercellular mitochondrial transport & enhances mesenchymal stem cell rescue efficacy
Tanveer Ahmad, Shravani Mukherjee, Bijay Pattnaik, Manish Kumar, Suchita Singh, Manish Kumar, Rakhshinda Rehman, Brijendra K Tiwari, Kumar A Jha, Amruta P Barhanpurkar, Mohan R Wani, Soumya S Roy, Ulaganathan Mabalirajan, Balaram Ghosh, Anurag Agrawal
DOI: 10.1002/embj.201386030 |Published 15.01.2014
Abstract
There is emerging evidence that stem cells can rejuvenate damaged cells by mitochondrial transfer. Earlier studies show that epithelial mitochondrial dysfunction is critical in asthma pathogenesis. Here we show for the first time that Miro1, a mitochondrial Rho‐GTPase, regulates intercellular mitochondrial movement from mesenchymal stem cells (MSC) to epithelial cells (EC). We demonstrate that overexpression of Miro1 in MSC (MSCmiroHi) leads to enhanced mitochondrial transfer and rescue of epithelial injury, while Miro1 knockdown (MSCmiroLo) leads to loss of efficacy. Treatment with MSCmiroHi was associated with greater therapeutic efficacy, when compared to control MSC, in mouse models of rotenone (Rot) induced airway injury and allergic airway inflammation (AAI). Notably, airway hyperresponsiveness and remodeling were reversed by MSCmiroHi in three separate allergen‐induced asthma models. In a human in vitro system, MSCmiroHi reversed mitochondrial dysfunction in bronchial epithelial cells treated with pro‐inflammatory supernatant of IL‐13‐induced macrophages. Anti‐inflammatory MSC products like NO, TGF‐β, IL‐10 and PGE2, were unchanged by Miro1 overexpression, excluding non‐specific paracrine effects. In summary, Miro1 overexpression leads to increased stem cell repair.