
This graphic shows a matter wave hitting a 'Schrodinger's hat'. The intensity of the wave inside the device is magnified (red peak). Outside, the incident plane waves wrap around the device and re-join on the other side as if the device is not there. (Credit: Gunther Uhlmann, U. of Washington.)
Invisibility, the subject of magic, myth or imagination, is becoming reality. Mathematicians and others have been working on invisibility cloaks – perhaps not yet concealing Harry Potter's invisible sleeve, or Star Trek, the mythical Hermes/Perseus' helmet' but at least shielding small objects from detection by microwaves or sound waves. It started 'by mistake' in 2003 with inverse problems and breast cancer problems. The work is a type of inverse problem that uses measurements from the surface of a body — say a human body, or the Earth — to deduce what's inside. "This was the opposite of what we were looking for," Uhlmann recalled. "We were trying to make invisible things visible, and not the opposite." The group published its findings, including the mysterious case, in the journal Mathematics Research Letters. See story on the unexpected discovery.
The very first prototype of a cloaking device has been made by David Smith's group at Duke University.
The first invisible cloaks were microstates. Carpet cloaks were proposed by John Pendry, as a way of extending the operating range. Here an prototype article from 2006. And Ulf Leonhardt, also from 2006, in Science. Today there are many kinds of cloaks.
To make an object literally vanish before a person's eyes, a cloak would have to simultaneously interact with all of the wavelengths, or colors, that make up light. The visual appearance of objects is determined by the extent to which they modify light due to geometric scattering and material absorption. Thus, in order to hide the object from being detected, the invisibility cloak must conceal the changes to the light in both geometry and spectrum. Conventional optical components rely on gradual phase shifts accumulated during light propagation to shape light beams.
Leonhardt on invisibility: He gives more examples than these.
Why is light bent? Light rays obey Fermat's Principle of the least optical path. The refractive index n determines the optical length; distances in media with high n appear longer to light than distances in media with low n. When n varies, light rays try to stay as long as possible in regions of relatively low refractive index. In the mirage, light rays bend their paths to stay longer in the hot thin air above the desert where n is low.Fermat's Principle also explains Snell's law of refraction (discovered by the Arabian scientist Ibn Sahl more than a millennium ago). Refraction is familiar to everyone who has tried to gauge directions underwater. Imagine an angler sees a fish. Is the fish where it appears to be? No, because the water surface refracts the light coming from the fish (the sunlight reflected off the fish). The image of the fish reaches the eye of the angler at a different direction than the "line of sight". The angler is misled, unless he has learned from experience. Light is refracted at the water surface, because the refractive index n of air is smaller than the refractive index of water and, according to Fermat's Principle, light tries to stay as long as possible in a region of low n. In media where n gradually varies, like in the desert air above the hot sand, light is not refracted, but bent.
Another example is the green flash. When the Sun is setting the Sun's rays are bent in the atmosphere, because the air is thinner at higher altitudes and so the refractive index is lower there. The light rays are bent in the opposite way to a mirage (but for the same reason). So, when the Sun appears to be setting, she has already set, because of the light bending in the atmosphere. The refractive index n of air is larger for higher frequencies of light; blue or green light is refracted stronger than orange or red light, so blue or green light is more strongly bent. The blue light, however, is scattered in the atmosphere, coloring the sky blue, due to Rayleigh scattering (conjectured by Alhazen of Basra during the early eleventh century and by Leonardo da Vinci ca. 1500). Therefore, the last light from the setting Sun is green and appears as a green flash when the atmospheric conditions are right.A new solution to the problem of invisibility based on the use of dielectric (nonconducting) anisotropic metamaterials. Our optical cloak not only suggests that true invisibility materials are within reach, it also represents a major step towards transformation optics.
One system employs the natural birefringence of the calcite. In such materials the refraction of light depends on the polarization. Today also graphene has been used for achieving spatial patterns, which by manipulating the graphene also can be changed. "by designing and manipulating spatially inhomogeneous, nonuniform conductivity patterns across a flake of graphene, one can have this material as a one-atom-thick platform for infrared metamaterials and transformation optical devices. Varying the graphene chemical potential by using static electric field yields a way to tune the graphene conductivity in the terahertz and infrared frequencies. Such degree of freedom provides the prospect of having different “patches” with different conductivities on a single flake of graphene."
Leonhardt, cont.


Imagine the material appears to move the points of space to different locations:
it performs a coordinate transformation
from a virtual space (A) to physical space (B).
It creates the illusion that light flows through the empty virtual space (A),
whereas in reality it propagates in (B).
In physical space, one point of virtual space has been expanded to finite size.
As light would not notice a single point it flows around the expanded region
without any distortion.
So the interior of the expanded point is hidden
and the act of hiding is concealed.
In short, the material makes a cloaking device. An optical medium changes
the measure of distance perceived by light.
Suppose that the medium preserves the right angles
between light rays and wavefronts like the
Mercator Projection preserves the angles between
longitude and latitude.
In this case, the medium
could smoothly guide light without reflection.
In mathematics, maps that preserve angles are known as
conformal maps
and their optical implementation
with an appropriate refractive-index profile is an
Optical Conformal Mapping.
Most conformal maps require more then one sheet
to represent them, but rather several or even infinitely many,
sewn together at branch cuts between branch points.
Some generate mind-boggling
Riemann surfaces
like the one
that represents the tiling on the title page
of the book on
complex analysis
shown below.
The picture beside the book shows the tiling
behind the scenes of the figure on light propagation.
Riemann surfaces have inspired some of
M.C. Escher's intriguing paintings.
Their many branches can be used to hide
regions of space, provided an
optical medium facilitates the mapping.
If one has something to hide, one should hide it
on Riemann sheets. Light that has passed the branch cut to another sheet
of the optical Riemann surface will get stuck there
and never return.

From Leonhardts page. Remarcable! Go to his page for more ref.
- U. Leonhardt, Optical Conformal Mapping, Science Express, May 25 (2006).
- U. Leonhardt, Notes on Conformal Invisibility Devices, New Journal of Physics 8, 118 (2006).
- A. Hendi, J. Henn, and U. Leonhardt, Ambiguities in the Scattering Tomography for Central Potentials, Physical Review Letters 97, 073902 (2006).
- U. Leonhardt and T. G. Philbin, General Relativity in Electrical Engineering, New Journal of Physics 8, 247 (2006).
- T. Ochiai, U. Leonhardt, and J. C. Nacher, A novel design of dielectric perfect invisibility devices, J. Math. Phys. 49, 032903 (2008).
- U. Leonhardt and T. Tyc, Broadband Invisibility by Non-Euclidean Cloaking, Science Express, November 20 (2008). For downloading a free copy click here.
Macrostates hidden.
Now they electromagnetically hide objects that can be seen with the naked eye and do so at visible wavelengths, so called optical transformations. To build such a cloak you need a material that will bend the incoming and outgoing light rays by different amounts — determined by the dimensions of the object underneath. The cloaks are also relatively cheap and easy to make, being constructed from the natural material as calcite, the refractive index of which depends on the relative orientation of an incoming light wave’s polarization axis and the calcite’s optical axis. Calcite is perfect for the job because the speed at which polarized light passes through it depends on the crystal's orientation. So by sticking together two pieces of crystal, it is possible to create a cloak that bends incoming and outgoing light by the desired amount. See Fooling an observer.
- See Macroscopic Invisibility Cloak for Visible Light by Baile Zhang (also this), Yu Luo, Xiaogang Liu, George Barbastathis 2010, on the broad range of wavelengths visible to the human eye, and Macroscopic Invisibility Cloaking of Visible Light by Xianzhong Chen, Yu Luo et al. 2010.
All the invisibility cloaks demonstrated thus far, however, have relied on nano- or micro-fabricated artificial composite materials with spatially varying electromagnetic properties, which limit the size of the cloaked region to a few wavelengths. Here we report realisation of a macroscopic volumetric invisibility cloak constructed from natural birefringent crystals. The cloak operates at visible frequencies and is capable of hiding three-dimensional objects of the scale of centimetres and millimetres.From the work of Yu Luo et al. carpet cloaks can be built from homogeneous – rather than more complex inhomogeneous – materials, as long as those materials are anisotropic.
These orientations were fixed such that lightwaves with a given polarization that bounce off a wedge-shaped object placed underneath the cloak emerge travelling in the same direction and at the same height that they would have done had they bounced straight off the mirror beneath the object. The wedge, having a base length, width and height of 38 mm, 10 mm and 2 mm respectively, can easily be seen with the naked eye.
Whirlpools and invisibilities. A hole carved out of space–time, a pocket in reality, or a chicken crossing the road. Carpet cloaks render covered objects invisible by bending light rays as they enter the cloak and then when they exit it — after they have bounced off the hidden object. The light is deviated in such a way that the rays seem to have been reflected directly from the ground underneath the object — as though the object was not there. Invisibility of macroscopic objects is a reality at last, says a Nature article.
Today they can also make cloaks that flexes with temperature. See Design of an optical reference cavity with low thermal noise and flexible thermal expansion properties. And topological transitions by manipulating the photonic environment and photonic density. They describe a transition in the topology of the iso-frequency surface from a closed ellipsoid to an open hyperboloid by increased rates of spontaneous emission of emitters positioned near the metamaterial.
New degrees of freedom are attained by introducing abrupt phase changes over the scale of the wavelength. A two-dimensional array of optical resonators with spatially varying phase response and subwavelength separation can imprint such phase discontinuities on propagating light as it traverses the interface between two media. Anomalous reflection and refraction phenomena are observed in this regime in optically thin arrays of metallic antennas on silicon with a linear phase variation along the interface, which are in excellent agreement with generalized laws derived from Fermat’s principle
Another sytem is anomalous localised resonance. Stealth technology is designed to make objects of military interest as black as possible to radar. Here the first line of defence is to reflect incoming radar waves off at odd angles. The waves that reach the object are then absorbed without reflection using impedance matching. They disappear without any echo detectable by radar. Perfect invisibility devices with isotropic media are proven to be impossible due to the wave nature of light. No illusion is perfect, or, expressed in mathematical terms, the inverse scattering problem for linear waves in isotropic media has unique solutions. But the imperfections of invisibility might be invisibly small. Maybe the device would create a slight haze, instead of a perfect image
A group has built a cylindrical “invisibility cloak” that shields objects from water waves by directing those waves around the object as if it weren’t there. Wikipedia:
Janos Perczel, 22, an undergraduate student at St Andrews University in Fife, has developed an optical sphere which could be used to create an "invisibility cloak". He said that by slowing down light by way of an optical illusion, the light can then be bent around an object to "conceal" it. Attempts have already been made to create invisibility cloaks but research shows that efforts are limited because any cloak would only work within certain backgrounds. But by slowing down the rays of light, Mr Perczel says the cloak wearer can move around ever-changing backgrounds.
Mr Perczel, from Hungary, came up with the idea under the guidance of "invisibility expert" Professor Ulf Leonhardt at the university's school of physics and astronomy. The student recognised the potential of the invisible sphere and spent eight months fine tuning his project. The key development lies in the ability of the sphere, an optical device, to not only remain invisible itself but to slow light.
According to Prof Leonhardt, all optical illusions can slow down rays of light and the sphere can be used to bend this illusion around an object, reflecting off it and making it appear to be invisible. Mr Perczel added: "When the light is bent it engulfs the object, much like water covering a rock sitting in a river bed, and carries on its path, making it seem as if nothing is there. Light however can only be sped up to a speed faster than it would travel in space, under certain conditions, and this restricts invisibility cloaks to work in a limited part of the spectrum, essentially just one colour. This would be ideal if somebody was planning to stand still in camouflage. However, the moment they start to move, the scenery would begin to distort, revealing the person under the cloak. By slowing all of the light down with an invisible sphere, it does not need to be accelerated to such high speeds and can therefore work in all parts of the spectrum." See "Student makes 'invisibility cloak'". Belfast Telegraph. 9 August 2011. Leonhardt, Ulf; Smith, David R (2008). "Focus on Cloaking and Transformation Optics". New Journal of Physics 10 (11): 115019.So, bending light can be illusory? That we have known for some time. Gravitational lenses use the same technique? They can even reveal hypothetical dark matter stars? See also wikipedia articles:
Invisibility in 3D.
Hiding a realistic object using a broadband terahertz invisibility cloak by Fan Zhou, Yongjun Bao, Wei Cao, Colin Stuart, Jianqiang Gu, Weili Zhang, and Cheng Sun. Scientific Reports 1 2011. Here Tolga Ergin, Karlsruhe Institute of Technology in Germany popular in BBC, based on the concept that you can "transform space" with a material. The carpet cloak was originally designed to work in two dimensions. There has been difficulties in fabricating cloaking devices that are optically large in all three dimensions. The Karlsruhe team used a technique called laser writing to create their 3-D cloak. This uses a very finely focused laser, to "write" into a light-sensitive material.
"Wherever you put the focus spot into the material, it will harden," explained Dr Ergin. "It's a similar process to photography - when you develop it, whatever hasn't been exposed to the laser will be washed away." Fan Zhou et al. in Nature offers a technical feasible solution for designing and fabricating 3D THz transformation optics :
The first experimental demonstration of a 3D THz cloaking device fabricated using a scalable Projection Microstereolithography process, is presented. The cloak operates at a broad frequency range between 0.3 and 0.6 THz, and is placed over an α-lactose monohydrate absorber with rectangular shape. Characterized using angular-resolved reflection THz time-domain spectroscopy (THz-TDS), the results indicate that the THz invisibility cloak has successfully concealed both the geometrical and spectroscopic signatures of the absorber, making it undetectable to the observer.Spectra maps of four experimental cases. From the Nature paper.
In this case, the researchers use the device to cloak a bump one micrometre (one thousandth of a millimetre) high. "But in theory there are no limits [to the size of the object you could hide]", said Dr Ergin. "You could blow this up and hide a house." (I guess that is adressed to the fond money keepers?)
"Photonic crystals usually work because the constitutive elements are not visible to the wavelength by which one observes them," Professor Hess explained."So if you look at the desk in front of you, you don't see the individual atoms because they are so small. You just see whole structure - the wood or the plastic."This means that cloaking devices for visible light would have to be made up of much smaller rods. So for this technique, the laser beam would have to be made even smaller.Currently, the rods can be made as small as 200 nanometres. To hide a bump from visible light would require rods as small as 10 nanometres.
- J. Fischer, T. Ergin, and M. Wegener, “Three-dimensional polarization-independent visible-frequency carpet invisibility cloak”, Optics Letters, in press.
Plasmonic invisibility effects
Plasmon is waves of electrons in metals. " ... may induce a dramatic drop in the scattering cross-section, making the object nearly invisible to an observer," Achieving transparency with plasmonic coatings, by Andrea Alu, Nader Engheta 2005. You've seen cloaking technology at work on television, when blue backgrounds are used to make a person invisible. A human could be made impossible to detect in longer-wavelength radiation such as microwaves, but not from visible light. A spaceship might be made transparent to radio waves or some other long-wavelength detector. Here we see how a proper design of these lossless metamaterial covers near their plasma resonance may induce a dramatic drop in the scattering cross section, making the object nearly invisible to an observer.
The earlier article: Extremely Low Frequency Plasmons in Metallic Microstructures, JB Pendry, I Youngs, AJ Holden and WJ Stewart, Phys. Rev. Lett. 76 4773-6 (1996).
Ultra-compact On-Chip Plasmonic Light Concentrator, by Ye Luo, Maysamreza Chamanzar, Ali Adibi, 18 Apr 2012 present
a novel approach for achieving tightly concentrated optical field by a hybrid photonic-plasmonic device in an integrated platform, which is a triangle-shaped metal taper mounted on top of a dielectric waveguide. This device, which we call a plasmomic light concentrator (PLC), can achieve side-coupling of light energy from the dielectric waveguide to the plasmonic region and light focusing into the apex of the metal taper (at the scale ~10nm) at the same time. For demonstration, we numerically investigate a PLC, which is a metal (Au) taper on a dielectric (Si3N4) waveguide at working wavelengths around 800nm. We show that three major effects (mode beat, nanofocusing, and weak resonance) interplay to generate this light concentration phenomenon and govern the performance of the device. By coordinating these effects, the PLC can be designed to be super compact while maintaining high efficiency over a wide band. In particular, we demonstrate that under optimized size parameters and wavelength a field concentration factor (FCF), which is the ratio of the norm of the electric field at the apex over the average norm of the electric field in the inputting waveguide, of about 13 can be achieved with the length of the device less than 1um for a moderate tip radius 20nm. Moreover, we show that a FCF of 5-10 is achievable over a wavelength range 700-1100nm when the length of the device is further reduced to about 400nm.
Cloak allows objects to move undetected, in hidden ways.
The latest development, by Martin McCall et.al. might see cloaks add yet another dimension to their capability: time, in ‘Space-time cloak’ to conceal events, 2010. He has mathematically extended the idea of a cloak that conceals objects to one that conceals events.
Scientists have developed a recipe for manipulating the speed of light as it passes over an object. WAU. Can that be true?
McCall:
The idea is to create a tunnel through which an object could perform an action – move or change shape, f. ex. – while appearing as doing nothing at all. The cloak could also find uses in signal processing: a detector placed inside the cloak would be able to "pause" a signal travelling through the wall while it first deals with a signal passing through the tunnel. When the leading edge of a light wave hits the cloak, the material is manipulated to speed up the light, but when the trailing edge hits, the light is slowed down and delayed. "Between these two parts of the light, there will be a temporal void — a space in which there will be no illuminating light for a brief period of time,"The mathematics behind the invisibility cloak involves a geometric transform – which takes a point, inflates it and renders anything that lies inside the resulting bubble unreachable by the waves — true for water waves just as for electromagnetic waves. The object functions just like a whirlpool, and indeed generates the same solutions to the Navier-Stokes equations of fluid dynamics as a whirlpool does. Tornadoes also move things in unexpected manners.
- McCall MW, Favaro A, Kinsler P, et al, A spacetime cloak, or a history editor, JOURNAL OF OPTICS, 2011, Vol:13, ISSN:2040-8978 (publication doi) The cloak works by locally manipulating the speed of light of an initially uniform light distribution, whilst the light rays themselves always follow straight paths. Any 'perfect' spacetime cloak would necessarily rely upon the technology of electromagnetic metamaterials, which has already been shown to be capable of deforming light in ways hitherto unforeseen—to produce, for example, an electromagnetic object cloak. Nevertheless, we show how it is possible to use intensity-dependent refractive indices to construct an approximate STC, an implementation that would enable the distinct signature of successful event cloaking to be observed. Potential demonstrations include systems that apparently violate quantum statistics, 'interrupt-without-interrupt' computation on convergent data channels and the illusion of a Star Trek transporter.
- McCall MW, Favaro A, Kinsler P, et al, A spacetime cloak, or a history editor, J OPT-UK, 2011, Vol:13, ISSN:2040-8978 (doi)
- McCall MW, What is negative refraction?, JOURNAL OF MODERN OPTICS, 2009, Vol:56, Pages:1727-1740, ISSN:0950-0340 (publication doi) We review various published definitions associated with the phenomenon of negative phase velocity propagation of electromagnetic waves in meta-media, as observed through negative refraction.
- Kinsler P, Favaro A, McCall MW, Four Poynting theorems, EUROPEAN JOURNAL OF PHYSICS, 2009, Vol:30, Pages:983-993, ISSN:0143-0807(publication doi) The Poynting vector is an invaluable tool for analysing electromagnetic problems. However, even a rigorous stress–energy tensor approach can still leave us with the question: is it best defined as E × H or as D × B? Typical electromagnetic treatments provide yet another perspective: they regard E × B as the appropriate definition, because E and B are taken to be the fundamental electromagnetic fields. The astute reader will even notice the fourth possible combination of fields, i.e. D × H. Faced with this diverse selection, we have decided to treat each possible flux vector on its merits, deriving its associated energy continuity equation but applying minimal restrictions to the allowed host media. We then discuss each form, and how it represents the response of the medium. Finally, we derive a propagation equation for each flux vector using a directional fields approach, a useful result which enables further interpretation of each flux and its interaction with the medium.
- (About Poynting's theorem. the general question of the physical interpretation of Poynting's theorem and compare two typical derivations of it. One of these uses the work done on a charge by an external electromagnet field, while the other considers the work done by the total field, external plus self-field).
Wormholes and magnetic monopoles?
Monopoles first received theoretical support when the brilliant theoretical physicist Paul Dirac showed mathematically that one possible reason for the quantization of the electric charge (in other words, why electric charge only occurs in integer amounts) is the existence of a complementary magnetic particle with a quantized magnetic charge. This put monopoles on fairly firm footing as far as quantum electrodynamics was concerned. When magnetic monopoles began popping up in grand unification theories as a consequence of the dissociation of electromagnetism, the strong force and the weak force, they gained even more adherents among theorists.
- A. Greenleaf, Y. Kurylev, M. Lassas, G. Uhlmann: Electromagnetic wormholes and virtual magnetic monopoles from metamaterials. Physical Review Letters 99, 183901 (2007) preprint.
- Claus Montonen and D. Olive, 1977. Magnetic monopoles as gauge particles? Cern.
Negative compressibility: Metamaterial would stretch when compressed, and vice versa, under any circumstances. New materials with 'negative compressibility': compress when they are pulled and expand when they are pushed. Metamaterials that do this have been built before. For example, vibrating aluminium bars with tiny cavities inside them create waves that oppose the push or pull applied. But the designs must be vibrated at just the right frequency to see the effect. Zachary Nicolaou and Adilson Motter of Northwestern University in Evanston, Illinois, have now designed a metamaterial that stretches when compressed, and vice versa, under any circumstances. 'What is interesting is that they study systems that are not responding to a vibration but to a steady applied force,' says John Pendry of Imperial College London. NewScientist article.
Based on a Nature article; Mechanical metamaterials with negative compressibility transitions. These destabilizations give rise to a stress-induced solid–solid phase transition associated with a twisted hysteresis curve for the stress–strain relationship. The strain-driven counterpart of negative compressibility transitions is a force amplification phenomenon, where an increase in deformation induces a discontinuous increase in response force.
Positively Negative, JB Pendry, Nature ‘News and Views’ 423 22-23 (2003)
Negative refraction = light would refract the other way, through a negative angle. a) Light incident on a normal material refracts at a positive angle (blue), but in a negative-index material the refraction angle is negative (red). Negative refraction occurs only in specially engineered materials. The response of a material to electric and magnetic fields is characterized by its permittivity, e, and its permeability, m, respectively.
It is easy to fabricate an artificial material with negative e using a lattice of thin metal wires. We then showed how to do the same thing for m: the required magnetic response was obtained from a lattice of metal ‘split rings’ that resonate with magnetic fields of a given frequency; the induced currents give a negative magnetic response. The third paper, by Smith and colleagues made the key advance. This team made a structure that combined these elements in a microwave experiment, the first demonstration of negative e and negative m in the same material. They went on to demonstrate negative refraction in their material.
Negative refraction = light would refract the other way, through a negative angle. a) Light incident on a normal material refracts at a positive angle (blue), but in a negative-index material the refraction angle is negative (red). Negative refraction occurs only in specially engineered materials. The response of a material to electric and magnetic fields is characterized by its permittivity, e, and its permeability, m, respectively.
It is easy to fabricate an artificial material with negative e using a lattice of thin metal wires. We then showed how to do the same thing for m: the required magnetic response was obtained from a lattice of metal ‘split rings’ that resonate with magnetic fields of a given frequency; the induced currents give a negative magnetic response. The third paper, by Smith and colleagues made the key advance. This team made a structure that combined these elements in a microwave experiment, the first demonstration of negative e and negative m in the same material. They went on to demonstrate negative refraction in their material.
The search for monopoles continues through experiments like the Monopole and Exotics Detector, or MoEDAL experiment, at CERN. This monopole is a singularity (a closed string?), but monopole could also be a duality with one pole shielded?
The solid-solid phase transition link contain headings like Challenges of Paradigm Building for Solid-State Transformations and Diffusional Transformations with Order-Disorder Transformations, phase separations and decomposition, magnetoresistence, time-resolvation, effect of pressure or strain on magnetic transitions etc.
A whirlpool or a wormhole? See
The team devised in 2007 wormholes in which waves disappear in one place and pop up somewhere else. Rays travel through the wormhole and emerge on the other end. "Invisibility research has led to discovery of 'wormholes'" ,
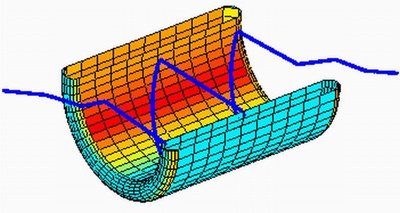
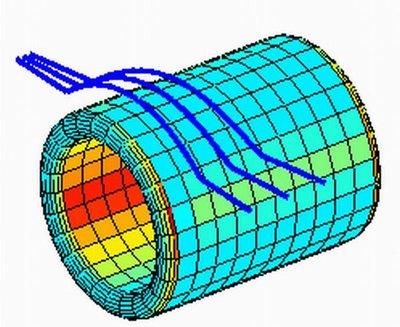
Their wormhole, named after the concept in general relativity, is a tunnel that would make light waves seem to disappear in one place and reappear somewhere else. "The idea is to create a tunnel of invisibility, a secret connection between two different points in space," Gunther Uhlmann said. It takes the invisibility concept from one dimension to two dimensions. "The wormhole comes from blowing up a line."
The wormhole discovery was covered in Nature, Scientific American and National Geographic.
Back to the Schrödinger hat!
"Schrödinger's hat," refer to the Schrödinger's cat in quantum mechanics. The name is also a nod to the ability to create something from what appears to be nothing, with L. Krauss words.
Gunther Uhlmann, (also doing inverse problems) Allan Greenleaf, Yaroslav Kurylev, and Matti Lassas at the University of Helsinki in Finland, all of whom are co-authors on the new paper.
- A. Greenleaf, Y. Kurylev, M. Lassas, G. Uhlmann: Schrodinger's Hat: Electromagnetic, acoustic and quantum amplifiers via transformation optics. Submitted PNAS, preprint
The team calls their modified invisibility cloak a Schrödinger's hat because tiny "parts" of waves or wavefunctions can be secretly stored, rather like a magician's hat, and detected. And the trick is that the rest of the wave would be scarcely changed. Outside the Schrödinger's hat the wavefunction would be "the old wavefunction multiplied by a constant, [which] may be very small". iInvisibility cloaks can be understood through an analogy with Einstein's general theory of relativity. This theory shows how very massive objects distort the underlying fabric of the universe, space–time. In the same way, certain man-made structures known as metamaterials distort an equivalent fabric, a virtual "optical space".
"You can isolate and magnify what you want to see, and make the rest invisible," said Uhlmann. "You can amplify the waves tremendously. And although the wave has been magnified a lot, you still cannot see what is happening inside the container."
"In some sense you are doing something magical, because it looks like a particle is being created. It's like pulling something out of your hat," he said. Matter waves inside the hat can also be shrunk, but concealing very small objects "is not so interesting."
The hat acting as invisible concentrators of waves. Compression! And expansion!
Sounds like mind-matter? Is mind a wormhole?
An artificial wormhole would work only for electromagnetic waves with a specified frequency. The wormhole device is just an optical device similar to a lens; not similar to space-time wormholes studied in general relativity, they point out.
The invisibility cloaking means coating of an object with a special metamaterial so that light goes around the object. The existing invisibility cloak uses tiny copper wires embedded in fiberglass panels to manipulate incoming waves.; from Invisibility cloaking and electromagnetic wormholes.

The team helped develop the original mathematics to formulate cloaks. Maxwell's equations have transformation laws that allow for design of electromagnetic parameters that would steer light around a hidden region, returning it to its original path on the far side. As waves hit the left side of the cloak they start to bend, so that waves leaving the cloak on the right appear as if they had never encountered an obstacle. In a paper, they solve equations showing how to make a cloak invisible to all incoming frequencies, including visible light. They also calculate how to shield living, glowing or electrically active objects.
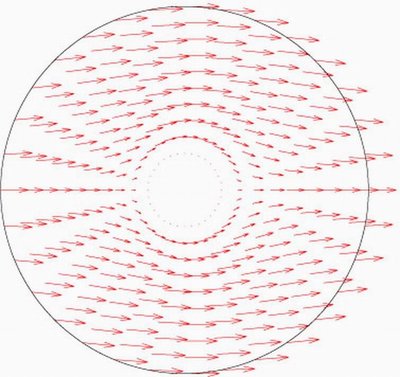
- "Full wave invisibility of active devices at all frequencies" to the journal Communications in Mathematical Physics. Coauthors are Allan Greenleaf at the University of Rochester, a longtime collaborator; Matti Lassas at the Helsinki University of Technology, a former post-doctoral researcher at UW; and Yaroslav Kurylev at Loughborough University in the UK. prove invisibility with respect to solutions of the Helmholtz and Maxwell’s equations, a singular transformation that pushes isotropic electromagnetic parameters forward into singular, anisotropic ones. We define the notion of finite energy solutions of the Helmholtz and Maxwell’s equations for such singular electromagnetic parameters, and study the behavior of the solutions on the entire domain, including the cloaked region and its boundary. Due to the singularity of the metric, one needs to work with weak solutions. Analyzing the behavior of such solutions inside the cloaked region, we show that, depending on the chosen construction, there appear new “hidden” boundary conditions at the surface separating the cloaked and uncloaked regions. We also consider the effect on invisibility of active devices inside the cloaked region, interpreted as collections of sources and sinks or internal currents. When these conditions are overdetermined, as happens for Maxwell’s equations, generic internal currents prevent the existence of finite energy solutions and invisibility is compromised...
- A. Greenleaf, Y. Kurylev, M. Lassas, G. Uhlmann: Inverse problems, invisibility, and artificial wormholes. Journal of Physics: Conference Series 124 (2008), 012005 (23pp) Proceedings of AIP 2007 First International Congress of Inverse Problems International Association.
A postdoctoral researcher working with Uhlmann, Hongyu Liu, published a paper showing that a wall could be made to appear when there is actually nothing there. Look for references on his page.
"It all comes from the same idea, making things expand and contract, making things look different things than what they really are."
Acoustic Cloaks.
"From the experimental point of view, I think the most exciting thing is how easy it seems to be to build materials for acoustic cloaking," Uhlmann said. Wavelengths for microwave, sound and quantum matter waves are longer than light or electromagnetic waves, making it easier to build the materials to cloak objects from observation using these phenomena.
- Acoustic cloaking theory, by A. Norris, Proc. R. Soc. A 8 September 2008 vol. 464 no. 2097 2411-2434, doi: 10.1098/rspa.2008.0076
Newly Developed Cloak Hides Underwater Objects from Sonar, 2011, demonstrated an acoustic cloak, a technology that renders underwater objects invisible to sonar and other ultrasound waves, a working prototype developed of metamaterial. "We are talking about controlling sound waves by bending and twisting them in a designer space," said Nicholas Fang. Fang's team designed a two-dimensional cylindrical cloak made of 16 concentric rings of acoustic circuits structured to guide sound waves. Each ring has a different index of refraction, meaning that sound waves vary their speed from the outer rings to the inner ones.
"Basically what you are looking at is an array of cavities that are connected by channels. The sound is going to propagate inside those channels, and the cavities are designed to slow the waves down," Fang said. "As you go further inside the rings, sound waves gain faster and faster speed." Since speeding up requires energy, the sound waves instead propagate around the cloak's outer rings, guided by the channels in the circuits. The specially structured acoustic circuits actually bend the sound waves to wrap them around the outer layers of the cloak.

The cloak offers acoustic invisibility to ultrasound waves from 40 to 80 KHz, although with modification could theoretically be tuned to cover tens of megahertz. "The geometry is not theoretically scaled with wavelengths. The nice thing about the circuit element approach is that you can scale the channels down while maintaining the same wave propagation technology."
- Shu Zhang, Chunguang Xia, Nicholas Fang. Broadband Acoustic Cloak for Ultrasound Waves. Physical Review Letters, 2011;
- V. M. García-Chocano, L. Sanchis, A. Díaz-Rubio, J. Martínez-Pastor, F. Cervera, R. Llopis-Pontiveros, J. Sánchez-Dehesa. Acoustic cloak for airborne sound by inverse design. Applied Physics Letters, 2011; 99 (7): 074102 DOI: 10.1063/1.3623761
Diffusional transformations.
'Thermal cloak' hides objects from heat', Sebastien Guenneau, et al. has proposed isolating or cloaking objects from sources of heat - essentially "thermal cloaking." An open-access journal Optics Express, taps into some of the same principles as optical cloaking and may lead to novel ways to control heat in electronics and, on an even larger scale, might someday prove useful for spacecraft and solar technologies.
Until now, he explains, cloaking research has revolved around manipulating trajectories of waves. These include electromagnetic (light), pressure (sound), elastodynamic (seismic), and hydrodynamic (ocean) waves. The biggest difference in their study of heat, he points out, is that the physical phenomenon involved is diffusion, not wave propagation.

"Heat isn't a wave - it simply diffuses from hot to cold regions," Guenneau, Institut Fresnel in France, says. "The mathematics and physics at play are much different. For instance, a wave can travel long distances with little attenuation, whereas temperature usually diffuses over smaller distances."
To create their thermal invisibility cloak, Guenneau &co applied the mathematics of transformation optics to equations for thermal diffusion and discovered that their idea could work. The researchers propose a cloak made of 20 rings of material, each with its own "diffusivity" - the degree to which it can transmit and dissipate heat. "We can design a cloak so that heat diffuses around an invisibility region, which is then protected from heat, or we can force heat to concentrate in a small volume, which will then heat up very rapidly."
This diffusion = wave was also found by Masao Nagasawa in the Schrödinger Equations and Diffusion Theory, 1993. Schrödingers wave equation can be used for diffusion.
The approach is fundamentally different from temperature-changing cloaks that heat and cool actively to mimic objects of different temperatures and have proven to "hide" a tank.
Quantum measurements.
In order to avoid perturbation and the decoherence from the observation of position, indirect methods of measurement have been developed.
Avshalom Elitzur and Lev Vaidman at Tel Aviv University in Israel pointed 1993 out that it is not always necessary to observe particles directly to learn something of their nature. The Elitzur-Vaidman bomb-tester paradox says: A BOMB triggered by a single photon of light is a scary thought. If such a thing existed in the classical world, you would never even be aware of it. Any photon entering your eye to tell you about it would already have set off the bomb, but you can also use quantum matter waves to detect a light-triggered bomb with light (interferometer, where a photon will take both paths at once and produce an interference pattern), In Elitzur and Vaidman's thought experiment, half the time there is a photon-triggered bomb blocking one path (see diagram). Only the real photon can trigger the bomb, so if it is the ghostly copy that gets blocked by the bomb, there is no explosion.
- Elitzur A. C. and Vaidman L. (1993). Quantum mechanical interaction-free measurements. Found. Phys. 23, 987-97. arxiv:hep-th/9305002
- Can Schrodinger's Cat Collapse the Wavefunction?, Keith Bowden 1997
- The Renninger negative-result experiment, see Mauritius Renninger
In 2000, Shuichiro Inoue, Gunnar Bjork and Jonas Söderholm of the Royal Institute of Technology in Stockholm, Sweden, used a technique involving two weakly interacting Bose-Einstein condensates, to show that you could get an image of a piece of an object without shining light on it. The concept of interference visibility can, and should, be generalized to describe weakly interacting multiply occupied coherent bosonic systems. There were no radiation damage to the tissue because no X-rays actually hit it. Visibility is not a good measure of a well-defined relative phase.
The 'hat' may be an easier way to perform such measurements, see the Schrödinger hat could spy on quantum particles. In the 'wormhole' not all the light passes around – often, a small amount will leak in. If the inside of the cloak had almost the same resonant frequency as that of the incoming light, say Uhlmann and colleagues, that wave's energy would build up, forming a localized excitation. This excitation behaves much like a particle, which the group has dubbed a "quasmon". This quasmon could then be released by making a slight alteration to the cloak's resonant frequency, perhaps through the application of a weak magnetic field. A little like 'tasting' the environment, to eavesdrop , like computer viruses do?
The potential of a Schrödinger's hat can be seen in the example of an electron in a box. Although the electron's wavefunction is spread throughout the box, a scientist may be able to guess the location of areas where it drops to zero. That scientist could then position a Schrödinger's hat at such a location, with no fear of the electron "noticing" the sensor's presence and collapsing into a definite state. If the experiment were to be repeated several times, the scientist might be able to map out where the electron definitely is not – and in doing so, learn something about where it actually is.
Leonhardt has shown us how to make artificial black holes, a video about Invisibility, levitate objects and even make things disappear. See also Perfect imaging, Fibre-optical black holes, Quantum levitation, Invisibility, Quantum catastrophes and Light in moving media.Ulf Leonhardt, at St Andrews Univ. in the UK, a member of Uhlmann's group, says that a device that works for microwaves could be made using circuit-board materials. A device for plasmons could be made from metal and plastic rings. He thinks a Schrödinger hat could even be developed for sound – allowing its users to eavesdrop on sound without disturbing it.
Anonymous matpitka@luukku.com said...
SvaraRaderaTo Ulla:
One TGD inspired variant of invisibility cloak would be based on phase transition transforming photons to dark photons at the surface of the invisible objects so that they would not be reflected nor absorbed. In this case one would however have a shadow. This would imply strange mirage like effects. An object in certain place would be seen it completely different position: image would travel along flux tubes.
If photons flow like hydrodynamical flow along the surface, even the shadow is absent. In TGD framework Maxwell's equations are replaced with conservation laws- essentially hydrodynamical equations- I have proposed that one obtains what could be called perfect hydrodynamical flow. This might give rise to perfect invisibility cloak.
comment on Mattis blog http://matpitka.blogspot.fi/2012/06/dark-magnetism.html#c1972295302033173237
A full varant of the Riemannian surfaces are found here
SvaraRaderahttp://www.sciencemag.org/content/323/5910/110.full.pdf?keytype=ref&siteid=sci&ijkey=AmRp24B6rB6O.
also given as a link in the text.
Much of the math behind described here http://arxiv.org/pdf/math/0611185v3.pdf
SvaraRaderahttp://www.kip.uni-heidelberg.de/matterwaveoptics/research/bosons/
SvaraRaderaBosonic quantum gases. Dark solitons.
Experimental Observation of Oscillating and Interacting Matter Wave Dark Solitons (Phys. Rev. Lett. 101, 130401 (2008))
Squeezing and entanglement in a Bose-Einstein condensate (Nature 455, 1216-1219 (2008))
The signal to noise ratio is limited by the counting statistics of independent particles (photons or atoms) which are propagating through the interferometer. Quantum mechanics, however, offers the possibility to overcome this classical limit using entangled states. Spin squeezed states, a special kind of entangled states, have theoretically been shown to improve the signal to noise ratio (SNR) in interferometers.
We experimentally observe spin squeezed entangled states in a Bose-Einstein condensate. The amount of spin squeezing in our system allows for an improvement of 3.8dB in the SNR of spatial interferometers.
To do so, we prepare the Bose-Einstein condensate in a 1-dimensional optical lattice with only a few sites occupied. Approximately 1000 atoms populate the sites in the center of the condensate. Slowly ramping up the lattice, slicing the condensate adiabatically into parts, evolves the state of the system towards a spin squeezed state. Even though the system is at a finite temperature we enter the entangled regime.
We observe the entangled states using sub shot noise site resolved atom number detection. Additionally we measure the relative phase of two wavefunctions peaked around neighboring sites of the lattice. Fluctuations in these conjugate variables provide a measure for fluctuations of a fictious spin which features the spin squeezing.
Quantum Interferometry
SvaraRaderawith Bose-Einstein Condensates
The project aims at the realization of a new class of interferometers based on entangled states of atomic Bose-Einstein condensates. These will have sensitivities beyond the shot-noise limit, potentially approaching the ultimate Heisenberg limit dictated by quantum mechanics. Our goal is to achieve an optimal control of the entanglement by means of novel experimental and theoretical tools to fully exploit its capabilities in precision measurements of time, forces, accelerations and electromagnetic fields. A successful completion of this project will disclose a new generation of quantum-enhanced sensors for applications and fundamental physics, and also lead to a better control and exploitation of quantum entanglement for ICT purposes. http://qibec.ino.it/
http://nextbigfuture.com/2012/05/mechanical-metamaterials-with-negative.html
SvaraRaderanegative compressibility transitions.
Plasmonic nanosensors with inverse sensitivity by means of enzyme-guided crystal growth
SvaraRaderaLaura Rodríguez-Lorenzo,
Roberto de la Rica,
Ramón A. Álvarez-Puebla,
Luis M. Liz-Marzán
& Molly M. Stevens
A way to use the results? But this is independent. Sensor amplifying!
because conventional transducers generate a signal that is directly proportional to the concentration of the target molecule, ultralow concentrations of the molecule result in variations in the physical properties of the sensor that are tiny, and therefore difficult to detect with confidence. Here we present a signal-generation mechanism that redefines the limit of detection of nanoparticle sensors by inducing a signal that is larger when the target molecule is less concentrated. The key step to achieve this inverse sensitivity is to use an enzyme that controls the rate of nucleation of silver nanocrystals on plasmonic transducers. We demonstrate the outstanding sensitivity and robustness of this approach by detecting the cancer biomarker prostate-specific antigen down to 10−18 g ml−1 (4 × 10−20 M) in whole serum.
The link http://www.nature.com/nmat/journal/vaop/ncurrent/full/nmat3337.html
SvaraRaderaMagnetic monopoles as gauge particles?, 1977.
SvaraRaderaC. Montonen,
D. Olive
http://dx.doi.org/10.1016/0370-2693(77)90076-4,
when quantized, the magnetic monopole soliton solutions constructed by 't Hooft and Polyakov, as modified by Prasad, Sommerfield and Bogomolny, form a gauge triplet with the photon, corresponding to a Lagrangian similar to the original Georgi-Glashow one, but with magnetic replacing electric charge.
And I found it on web. http://cdsweb.cern.ch/record/422232/files/CM-P00061677.pdf
These metamaterials are linked to the Casimir force. I did not write about it because it went too long already. Now there also seems to be a repulsive Casimir force!
SvaraRaderahttp://nextbigfuture.com/2010/12/repulsive-casimir-force-from.html
http://arxiv.org/abs/1006.5489
We examine a recent prediction for the chirality-dependence of the Casimir force in chiral metamaterials by numerical computation of the forces between the exact microstructures, rather than homogeneous approximations. We compute the exact force for a chiral bent-cross pattern, as well as forces for an idealized "omega"-particle medium in the dilute approximation and identify the effects of structural inhomogeneity (i.e. proximity forces and anisotropy). We find that these microstructure effects dominate the force for separations where chirality was predicted to have a strong influence. To get observations of chirality free from microstructure effects, one must go to large separations where the effect of chirality is at most sim10^{-4} of the total force.
http://arxiv.org/find/all/1/all:+AND+casimir+metamaterials/0/1/0/all/0/1
http://youtu.be/rJoAfMotMjU Mercedes invisible car: worldwide sensation.
SvaraRaderathanks to http://www.facebook.com/laborious.cretin
http://ow.ly/fwG19
SvaraRaderaInvisibility Cloaking by Coordinate Transformation
SvaraRaderaMin Yan, Wei Yan and Min Qiu
http://web.it.kth.se/~min/files/PIO_Cloaking.pdf
Department of Microelectronics and Applied Physics, Royal Institute of Technology (KTH), Electrum 229, 164 40 Kista, Sweden
http://phys.org/news/2013-01-ultrathin-invisibility-cloak-wide-applications.html Researchers have created a dc invisibility cloak made of a metamaterial that not only shields an object almost perfectly, but at 1-cm thick is also the thinnest cloak ever constructed, reaching the ultimate limit of thinness for artificial materials. As the first invisibility cloak that combines both near-perfect performance and extreme thinness, it could open the doors to practical applications. In the past, invisibility cloaks have been too large to be used in many real-world applications.
SvaraRaderahttp://apl.aip.org/resource/1/applab/v102/i1/p014102_s1?isAuthorized=no We propose and experimentally demonstrate an ultrathin but nearly perfect dc electric invisibility cloak. In the dc limit, transformation optics reduces to transformation electrostatics. Based on a special coordinate transformation, we derive a nearly perfect dc electric cloak which is composed of homogeneous and anisotropic conducting material. Due to the homogeneity feature, the dc cloak is realized using an ultrathin dc metamaterial with only one-unit-cell thickness, which is the ultra limit for practical artificial materials. Although ultrathin, our experimental results show that the dc cloak has excellent performance with nearly perfect cloaking behaviour.
http://www.materials360online.com/newsDetails/33860
SvaraRaderaMartin Maldovan of MIT has produced the theoretical framework that could make possible better control of heat flow in materials through the careful design of thermocrystals comprising alloys containing nanoparticles. This framework could lead to heat waveguides, heat lensing, thermal diodes, and thermal cloaking, among other long term potential applications.
“The theory outlines a completely new way of manipulating heat, when they created photonic crystals, it was a completely new way of manipulating light. Then, they created phononic crystals as a completely new way to manipulate sound.”
to transfer thermal energy flow from standard short wavelength particle transport to long wavelength wave transport-to make “heat behave like light,” according to Maldovan.
See also http://matterwave.physics.berkeley.edu/AtomInterferometry and http://matterwave.physics.berkeley.edu/LorentzInvariance
http://graviton.co.in/new-material-for-invisibility-cloaks/
SvaraRaderahttp://www.sciencenews.org/view/generic/id/350852/description/Light_breaks_up_to_cloak_gaps_in_time
SvaraRaderaA device that manipulates light to open up small gaps in time has crept toward implementation outside the lab. Detailed June 5 in Nature, it could soon improve security over fiber-optic lines or improve data streaming rates.
“It’s exciting to see this exotic manipulation of light and its applications for communications and data processing,” says Alexander Gaeta, a Cornell University physicist who demonstrated the first time cloak two years ago (SN: 8/13/11, p. 12).
A specially designed lens split light beams passing through a fiber into two segments. The trailing segment of light lagged behind the leading one by up to 50 trillionths of a second, creating a gap of total darkness between them. When Gaeta fired a laser at the fiber, the laser shot was undetectable because it had passed through the 50-picosecond interval of invisibility. Finally, Gaeta set up another lens to stitch the light segments back together, ensuring that the light beam emerged from the fiber looking exactly as it did at the start.
After reading Gaeta’s study, Joseph Lukens at Purdue University realized he could improve the technique. He designed an apparatus using off-the-shelf equipment that forced light to interfere and create repeating gaps of darkness at fixed temporal intervals. Each 40-picosecond gap was sandwiched between about 40 picoseconds of light, meaning that the time cloak was on roughly half the time.
Lukens’ study demonstrates how a time-cloaking device could eventually allow law enforcement or the military to prevent a nefarious person from communicating without the person’s realizing it. Just as the time gap in Gaeta’s experiment made the laser undetectable, the gaps created by Lukens’ cloak can conceal digital data. Lukens’ team tried to inject an electrical signal of 1s and 0s into the fiber, a task that would be no problem without a cloak, but the message never got encoded into the light beam. The receiver would assume that no message had been sent.
http://www.sciencenews.org/view/generic/id/349255/description/Sound_cloaks_enter_the_third_dimension
SvaraRaderaA simple plastic shell has cloaked a three-dimensional object from sound waves for the first time. With some improvements, a similar cloak could eventually be used to reduce noise pollution and to allow ships and submarines to evade enemy detection. The experiments appear March 20 in Physical Review Letters.
“This paper implements a simplified version of invisibility using well-designed but relatively simple materials,” says Steven Cummer, an electrical engineer at Duke University, who was not involved in the study. Cummer proposed the concept of a sound cloak in 2007.
our perception of the world depends on the scattering of waves. We can see objects because waves of light strike them and scatter.
José Sánchez-Dehesa, an electrical engineer at the Polytechnic Institute of Valencia in Spain, and his colleagues pursued a different method: Instead of preventing sound waves from hitting an object — in this case an 8-centimeter plastic sphere — they built a cloak to eliminate the scattered waves left in the sphere’s wake.
Using computer algorithms, the researchers came up with a design made up of 60 rings of various sizes that form a cagelike structure around the sphere. Simulations indicated that sound waves scattering off the sphere and the ringed cloak would interfere with each other and cancel out. (Noise-cancelling headphones exploit this phenomenon by emitting sound waves that minimize ambient sounds in a room.)
Because the cloak did not need to steer sound waves in complicated ways, Sánchez-Dehesa and his team built it out of plastic with the help of a 3-D printer. They hung their creation from the ceiling of an echo-free chamber, pointed a speaker at it and played a range of sound frequencies. For most frequencies, the sphere scattered an easily detectable amount of sound. But at 8.55 kilohertz — an audible high pitch — the cloaked sphere became imperceptible to the sensors behind it.
The study marks the first time scientists have ever cloaked a three-dimensional object from sound. That’s probably music to the ears of the U.S. Office of Naval Research, which partially funded the study to explore the possibility of sonar invisibility.
However, this cloak is just a small step toward stealth submarines. It has to be custom designed and built for each object, and it works only for a narrow frequency range coming from one direction. If the speaker had been set up anywhere else, the cloak would not have worked. Sánchez-Dehesa’s team plans to develop broadband and multidirectional cloaks.
But Cummer points out that even a limited cloak can have useful applications. He suggests that structures capable of manipulating a specific sound frequency from one direction could help minimize noise pollution from a congested highway. “The cloak does one thing quite well, with a very simple structure,” he says.
http://phys.org/news/2013-08-mathematician-event-cloaking-device-metamaterials.html
SvaraRaderahttp://shar.es/QudUR
SvaraRaderaTwo experiments show that metamaterials can shape the thermal distribution around an object, eliminating its disturbance of the thermal flux.
Now, writing in Physical Review Letters, two groups—Tiancheng Han from the National University of Singapore and colleagues, and Hongyi Xu and colleagues from Nanyang Technical University, Singapore—report independent experimental demonstrations of three-dimensional metamaterial “cloaks” for thermal flux—artificial covers capable of eliminating the disturbance created by an obstacle on the flow of heat [1, 2], potentially being able to improve, for instance, thermal imaging setups.
Beyond the challenges posed by optical waves, cloaking has been studied and proposed for sound, mechanical, seismic, and even matter waves [6, 7]. This has been possible because all these phenomena are governed by wave equations, in many ways analogous to the way electromagnetic signals and light propagate. In this context, one of the most popular cloaking approaches is based on coordinate transformation [2]: a gradual inhomogeneity in a suitably tailored metamaterial cover can be mapped into a curvilinear distortion of space in an equivalent homogeneous material. This implies that light rays, which follow straight lines in homogeneous media, can be bent at will around an object by transformation-based metamaterial cloaks, eliminating wave scattering and distortion [3].
Based on the invariance of the equation governing heat conduction under analogous coordinate transformations, Guenneau et al. [8] were able to extend this technique to propose a cloak able to manipulate the heat flux. What, effectively, is the job of such a thermal cloak? Heat is governed by diffusion and therefore does not propagate in wavelike form, it actually spreads smoothly away from the source. A difference in temperature between two regions of space induces a smooth temperature gradient from hot to cold as shown in Fig. 1(a). In the long run, this thermal diffusion tends to uniformly spread the temperature field.
If we now introduce a discontinuity, or an obstacle, within the flux path, as in Fig. 1(b), the isothermal lines become distorted. For instance, a good thermal conductor appears to repel the lines towards its surface, while an insulator attracts them. A thermal cloak hides objects from diffusive heat by smoothly guiding the thermal flux around them, so that mapping the isothermal lines outside the cloaked region would not reveal the presence of the discontinuity. This is achieved in Fig. 1(c), which shows the restoration of straight isothermal lines all around the cloaked object. Xu et al. [2] here mapped the temperature field all around the object of interest at a specific instant in time, proving full restoration of the original temperature distribution, as if the object were not there.
Review Article
SvaraRaderaThis article was invited by Masud Mansuripur.
Metamaterials are rationally designed man-made structures composed of functional building blocks that are densely packed into an effective (crystalline) material. While metamaterials are mostly associated with negative refractive indices and invisibility cloaking in electromagnetism or optics, the deceptively simple metamaterial concept also applies to rather different areas such as thermodynamics, classical mechanics (including elastostatics, acoustics, fluid dynamics and elastodynamics), and, in principle, also to quantum mechanics. We review the basic concepts, analogies and differences to electromagnetism, and give an overview on the current state of the art regarding theory and experiment—all from the viewpoint of an experimentalist. This review includes homogeneous metamaterials as well as intentionally inhomogeneous metamaterial architectures designed by coordinate-transformation-based approaches analogous to transformation optics. Examples are laminates, transient thermal cloaks, thermal concentrators and inverters, 'space-coiling' metamaterials, anisotropic acoustic metamaterials, acoustic free-space and carpet cloaks, cloaks for gravitational surface waves, auxetic mechanical metamaterials, pentamode metamaterials ('meta-liquids'), mechanical metamaterials with negative dynamic mass density, negative dynamic bulk modulus, or negative phase velocity, seismic metamaterials, cloaks for flexural waves in thin plates and three-dimensional elastostatic cloaks.
Muamer Kadic et al 2013 Rep. Prog. Phys. 76 126501 doi:10.1088/0034-4885/76/12/126501
Metamaterials beyond electromagnetism
http://iopscience.iop.org/0034-4885/76/12/126501/
http://www.dailymail.co.uk/sciencetech/article-2857281/The-invisibility-cloak-TIME-Experts-create-cloaking-device-capable-hiding-entire-events-bubbles.html
SvaraRaderaPlay Online Casino Site | Choegocasino Casino
SvaraRaderaOnline งานออนไลน์ Casino youtube mp3 Slots · Play'n Go · Play'n Go · Play'n Go · Play'n Go · Play'n choegocasino Go · Play'n Go. Play'n Go.